Lighting for houseplants – The complete guide
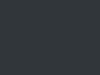
The second part of this blog series on lighting is all about practical comparisons and examples.
Are lamps really necessary?
Just like us, our plants have certain basic needs that must be met in terms of quality and quantity to enable healthy growth. In addition to the right substrate, moisture and nutrients, this is especially the proper light. Plants are photoautotrophic organisms, i.e. they meet their energy requirements exclusively by capturing light. If there is not enough light, growth stagnates and the plant can survive for a while on stored starch, for example, until it finally starves to death.
So we have to make sure that the light available to our plants can permanently cover their energy requirements. Diffuse room lighting is generally not sufficient for this, even what appears bright to our eyes is usually not enough for plant growth.
This article will discuss how much we really need in the end, how we can save costs and what else to look out for in terms of the quality of the plant lamp.
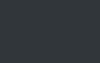
How much light do my plants need?
Lamps should be adequately dimensioned, but also cost money to purchase and operate. So you will often find a compromise in between. The actual light requirement depends on the plants being cultivated and is most easily expressed in lux. Lux is made up of the Si units lumen/square meter and is therefore very easy to calculate. Lumen is always the total amount of light emitted, in this case by our artificial light sources, and is indicated on the packaging.
Example:
The shelf of a Milsbo display cabinet (66 x 36 cm) is to be illuminated with a lamp with 1400 lumens: 1400 lumens / 0.24 m2 = 5830 lux
But is that a lot or very little? A few examples:
Sun under a clear sky: > 100,000 lux
Overcast sky in summer: 10-20,000 lux
Overcast winter day: ~2-5,000 lux
Trop. Rainforest in the undergrowth: ~2,000 lux*
Office lighting: 500 lux
Moonlight: approx. 0.1 lux
*from Becek & Salim 2017
Unshaded solar radiation near the equator can reach up to 200k lux (own measurement). However, we will never have to imitate such extreme values, as even plants adapted to strong light cannot utilize more than ~50k lux. For most of our typical house plants such as Alocasia, Philodendron or Begonia, however, significantly lower values are sufficient. In fact, they would burn or fade if they were exposed to so much light for so long. The following illustration provides a practical overview.
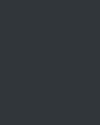
Fig. 1: Classification of various known houseplants into light requirement zones. The placements are intended rather as minimums, some plants can also cope flexibly over large areas.
The ~5000 lux from the example calculation is therefore in the middle range and is well suited for many well-known houseplants. For anthuriums or begonias, for example, it should be a little less, for candelabra flowers or desert roses more. Of course, this representation can only serve as a guideline and in many cases is intended as a minimum value. The amount of light required also depends on the spectrum and the duration of illumination; if the spectrum of the lamp is well matched, some reduction in lux can be achieved. A short 8h photoperiod, on the other hand, requires comparatively more light intensity than a 12h photoperiod.
The following illustration from our greenhouse shows that natural sunlight in our latitudes is often insufficient, not only in terms of intensity but also in terms of duration. The intensity of solar radiation on an average day in November 2023 is recorded behind double glass (similar to window glass) on a horizontal surface without shading. Significant lighting takes place from around 8 a.m. and sometimes reaches over 5000 lux at midday, but after 3 p.m. it is practically night for the plants. Plant lamps can supplement the missing intensity and/or duration effectively.
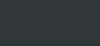
Fig. 2: Solar radiation in lux in our greenhouse in Herten, West Germany on a slightly cloudy day in November 2023. Measurement under double glass, horizontal surface without shading.
Practical tip: If you don’t want to buy an expensive luxmeter to measure light intensity at home, you can also use your smartphone. There are “Luxmeter” apps in the app stores for all operating systems that use the front sensor to measure brightness. Of course, this is not very accurate, but it is more reliable than the human eye and sufficient for general assessments.
What light do plants need?
For millions of years, plants have only ever had one source of light – the sun. Accordingly, evolution has ensured that precisely this light can be used as efficiently as possible. So basically, you can do nothing wrong if you use artificial lighting that is as similar to sunlight as possible, i.e. white light. However, due to various effects of the absorption properties of the pigments in the plant tissue and of optoelectronics to imitate the desired light, there are also many possibilities for optimizing the spectrum.
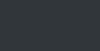
Fig. 3: Comparison of the photosynthetic action spectrum according to McCree, K. J., 1972 (modified) with marking of PAR radiation and weighting of lumen
The graphical representation of the spectrum of the light source is necessary for assessment. It simply describes the brightness of the light source in the different colors, specified in nanometers of the wavelength of the electromagnetic radiation. Photosynthesis takes place in the range of approx. 400 – 700 nm (PAR), which actually corresponds pretty closely to our ability to see. Shorter-wave radiation, i.e. < 400 nm, is UV radiation that has no direct significance for photosynthesis, but can certainly support the formation of secondary metabolites. In plants, for example, these can be protective pigments (anthocyanins) that give leaves a reddish color. In private culture, however, this is usually ignored. After UV comes violet and then blue radiation up to approx. 500 nm. Blue light is essential for photosynthesis, chlorophyll has absorption maxima at approx. 430 and 450 nm. Between 500 and 600 nm is green to yellow, this range is also important for photosynthesis. Plants appear green because a slightly larger proportion of the green light is reflected, but the majority of the radiation is utilized by carotenoids and other so-called antenna pigments of the light-collecting complex of higher plants. Green radiation also penetrates deeper through the upper leaf layer (Bugbee, B., 2016). Above 600 nm wavelength comes orange and above 650 nm red, this radiation is also essential for photosynthesis, chlorophyll again has absorption maxima at approx. 640 and 660 nm. Above 700 nm, near-infrared radiation begins; we can no longer see this light. It is also no longer absorbed by chlorophyll, but this light still has important functions (more on this later).
What kind of light do LED lamps produce?
Light-emitting diodes are currently superior to all other artificial light sources in terms of efficiency and light quality, so other technologies such as HID, fluorescent lamps etc. no longer need to be considered for hobby grow lights. LEDs initially only emit a monochromatic, i.e. single-color light with a bandwidth of approx. 10 nm, e.g. 660 nm +-5 nm. This is also used directly for blue and red in order to provide the most light at the strongest absorption peaks of the plant pigments.
If you choose to stick with it, you get the violet light familiar from many “plant lamps”. It looked very characteristic and was therefore a simple marketing argument that plants needed just such lamps. However, this has already been known better since at least the 1970s with McCree’s measurements. Nevertheless, the information persists.
White LEDs generate light by converting blue light of 450 nm into all other wavelengths using a yellow phosphor layer. The quality of the blue base LED and the phosphor mixture determines the values such as efficiency, color temperature or color rendering (CRI).
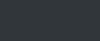
Fig. 4: Spectrum of the Jungle Lux Growlight in the range of 400 – 800 nm
Natural, neutral light has a color temperature of approx. 4000 Kelvin. This appears neither yellowish nor too cool white and is also an ideal mid-range value for the plants. This value is achieved by balancing the total amount of all red and blue light components. 3000K or 5000K are also good alternatives.
Another quality feature is the color rendering, a high CRI value of up to 100 indicates a high level of sunlight resemblance. Gaps in the spectrum cause deductions in the CRI, colors are then underrepresented. Cheap lamps often have values around 70 CRi, while values around 80 are sufficient for room and plant lighting. Even better values > 90 only play a role for e.g. photography.
Pure white LEDs may already be sufficient as plant lamps. The blue peak is usually sufficiently strong, but in the red range there is a lack of intensity in the longer wavelength range > 650 nm. This is due to technical reasons, as no phosphorus has yet been developed that would have a maximum in this range. To solve this problem, some plant lamps have extra red LEDs in addition to the white ones to increase efficiency. This is clearly visible in the spectrum.
Some high-end growlights also have individual far-red diodes built in, which address the phytochrome system of the plants in the range above 700 nm, which has further positive effects.
Efficiency and power consumption
In addition to the quality of the light, we would of course also like to address the light intensity already mentioned. The more efficiently the lamp works, the less electricity we need to draw.
Depending on the manufacturer, different values may be specified. The best known is the lumen, which is also the basic unit for the lux standard. This is usually a good reference value, but it is a heavily weighted value, as shown in Fig. 3. Lumen is based on the sensitivity of the human eye, which is highest at 555 nm. Here, even low intensities produce high lumen values, although this radiation is actually somewhat less efficient for plants. If you compare similar light sources with each other, you can still make a good comparison with lumen/lux. However, manufacturers sometimes cheat when it comes to brightness by mixing in extra green for high lumen values.
The efficiency of the lamp is specified in lumen/watt.
Example: Jungle Lux 1400 Lumen / 9 Watt = 155 Lumen/W (Inkl. Netzteil)
If an external power supply unit is required for operation, you will have to add another ~10% to the watts.
(The consumption of Far Red or UV diodes should theoretically be subtracted from the watts, but that makes it a bit too complicated)
A more independent value is the PAR value, which is defined as µmol/s (total light as lumen) or µmol/Joule (efficiency as lumen/W), but is often not specified for many lamps. The units of the values may look rather abstract, but they are similar to the more familiar lumens, only from the perspective of plants. Unfortunately, this value also does not allow any conclusions to be derived about the quality of the spectrum. For example, a pure red lamp can have a very high PAR value, but is useless if the other spectra are missing. With a known, balanced spectrum, however, the PAR value is a useful indication.
The so-called PPFD value is rather difficult to interpret in terms of efficiency, as it depends on the measuring distance and, in particular, the housing of the lamp.
In the Jungle Lux example, the 1400 lumens correspond to around 21 µmol/s and the 155 lumens/W to around 2.2 µmol/J. Higher values are better, lower values are worse. Again, neither UV nor infrared values are taken into account.
Practical examples
In fact, there are extreme differences in the efficiency of the lamps available on the market. Some currently produced models do not even reach 50 lumen/W, which is less than with old T5 or T8 tubes. It is hard to understand how something like this can happen…
The best high-end models achieve up to 200 lumen/W, so within LED technology there is a factor of 4 difference in how much electricity can be saved. A good 10 W LED lamp can emit just as much usable light as an unsuitable 40 W LED lamp.
At 12 hours a day, that makes:
30W * 12 * 365 = 131.400W per year;
131,4 kWh * 0,4€/kWh = 52,56€
So replacing this one example lamp saves over €50 per year at current electricity costs (Germany 2023).
Tab. 1: Comparison of some plant lamps according to efficiency (performance data include power supply unit)
Modell | Price (11.23) | Power (W) | Lumen | Efficiency (Lumen/W) | PPF (μmol/s) | Efficiency (μmol/J) | Note |
Sanlight Flex II | 40,20 + 22,08 | 11 | ~1900? | 173 | 25 | 2,30 | Without Red/Far Red |
Jungle Lux 60 FR | 34,99 | 9 | 1400 | 155 | 21 | 2,21 | With Red/Far Red |
Jungle Lux Spot | 29,99 | 12 | 1500 | 125 | 23 | 1,95 | With Red/Far Red |
Philips LED Reflector 2700K | 7,99 | 6 | 640 | 106 | – | – | Good budget spot |
Eheim classic LED daylight | 49,85 | 7,7 | 810 | 105 | – | – | Aquarium light |
Sansi | 29,99 | 36 | 3250 | 90 | 66 | 1,83 | High heat build-up |
Baltimore LED-Spotlight | 9,95 | 15 | 1180 | 52 | – | – | Simple construction spotlight |
Chihiros WRGB II Slim | 149,99 | 23 | 1200 | 77 | – | – | Popular aquarium lamp |
DOOA Magnet Light G | 79,99 | 11 | 475 | 43 | – | – | Why? |
The Sanlight and Jungle Lux from the examples are linear lights, these have a high beam angle and therefore very uniform illumination even at low distances. The distance to the plants should be between approx. 20-100 cm. Areas of application include display cabinets, shelves, growtents, terrariums and aquariums.
On the other hand, lamps such as the Sansi or the Jungle Lux spot are spotlights that emit light in a focussed manner from a rather point source. They are used to illuminate plants that are a little further away, but illuminate a slightly smaller area. Typical distances are between 50-200 cm, depending on the power.
Lifetime
LEDs can last a very long time with a proper thermal management. This is why the chips are usually permanently installed and cannot be replaced like light bulbs. An operating life of 10 years at 12 hours a day is certainly realistic and even then the lamp is not yet defective, but has only lost light output.
However, this only works with sufficiently dimensioned heat sinks in the lamp. Some manufacturers make savings here, sometimes a heat sink is even missing completely or is designed as a plastic dummy (a rogue who thinks evil). Such models lose a lot of light intensity after just a few months or fail completely.
Ideally, it should be possible to touch a lamp with your hands without burning yourself, even in continuous operation. For small spots with high output, the temperature at the heat sink may be above 50°C, but the cooler the better.
Far Red and morphogenesis
Photomorphogenesis describes the light-dependent shape of plants. In addition to chlorophyll for energy production, all higher plants have other, less known light-collecting pigments that can provide them with information about the quality of light. In this way, the plants practically “see” their surroundings and adapt their growth accordingly.
One of the most important of these systems is the phytochrome complex, which specifically perceives radiation at around 730 nm wavelength (Far Red) and sets it in relation to the available red radiation (660 nm) (Durazzo, B. D., 2021).
But why does this give the plant an advantage in being able to detect near-infrared radiation?
The answer to this question could be particularly important for our jungle plants such as Alocasia, Philodendron, Begonia or Selaginella.
In nature, these plants are not usually found in direct sunlight, but on the forest floor under a dense canopy of leaves. This already filters out a large part of the red and blue radiation before the light reaches the plants further down. The light that is still available is therefore shifted into the green. How much shade the plants are exposed to is important information, because leaf size or chlorophyll synthesis, for example, depends on it (Stutte, G. W., 2009). If there is a lot of shade, large, dark leaves must be used to capture as much remaining light as possible, whereas if there is a lot of natural sunlight, they would quickly burn.
This is precisely why plants use Far Red light, as it penetrates the leaf canopy to a greater extent than normal red light. If the proportion of Far Red to Red is significantly higher than normal, the plant thinks it is being shaded by others and grows differently as a result.
In lettuce (Lactuca sativa), adding small amounts of Far Red to the lighting has already increased the biomass by over 50%, with otherwise the same light intensity (Wenqing et al. 2021).
Of course, our blue iridescent plants that we have discussed in this blog article are also from the shade zones of tropical rainforests and tend to receive particularly high levels of Far Red radiation in these locations. An intensifying effect of Far Red radiation on the blue coloration would therefore be expected. In fact, exactly this has already been shown for Selaginella uncinata at approx. 1000 lux and an R:FR ratio of 0.35 (Hebant & Lee, 1984) and is also to be expected for other iridescent plants.
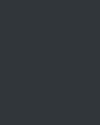
Fig. 5: Selaginella uncinata shows a blue iridescence as an adaptation to shady locations
Summary
- Know the light requirements of the cultivated plants (e.g. from Fig. 1)
- For existing setups, measure with a luxmeter (also available as a mobile app)
- Determine the amount of light required, e.g. in lumens, according to area and light requirement
- Comparing is worth twice as much when buying lamps (examples in Tab.1)
- Select LED lamps with white light and high efficiency > 120 lumen/W
- Plant-optimized spectra with high CRI, extra red and far red have further positive effects
All images in this article, unless otherwise stated, and text: © Nils Schmitz